
In the lithium-ion battery industry and innovation space, the government and the public desire to improve environmental, sustainability and governance (ESG) within the battery value chain. In the EU, upcoming changes to the Battery Directive are guiding that narrative(European Union, 2021). Although the United States does not require any non-lead acid recycling by law, the recent EPA national recycling plan will seek an overall recycling rate of 50% by 2030(EPA, 2021). Whereas the EU rule will require a 65% LIB recycling rate by 2025 and a minimum recycled content of new lithium-ion batteries, no similar requirement is pending in the US.
The challenges to implementing the EU directive are technical. Specifically, the currently constructed commercial recycling technologies cannot achieve the required recycling rate using pyrometallurgical technology(Umicore, 2021). Using pyrometallurgical processing, the graphite, plastic separator, the binder, and electrolyte are combusted which collectively represent ~42% of the weight of the battery(Jacoby, 2019). In addition, aluminum from the current collector and manganese & lithium from the cathode report to the slag from pyrometallurgical processing (Sommerfeld et al., 2021). The slag may be used for aggregate or but further processing strategies to recover lithium have not yet proven to be economic.(Rajaeifar et al., 2021). Pyro processing causes at least 50% of the weight of the battery unavailable for reuse into the battery value chain(Cheng et al., 2021). When a representative from Umicore was queried regarding how the Hoboken, BE recycling facility would meet the upcoming EU Battery Directive, his given answer was the company “needs to establish technology to meet requirements”(Battery Associates, 2021). It is not possible to meet those requirements if ~ 42% is combusted at 1400 ⁰C. Even if innovations can recover lithium, manganese, and aluminum from the slag, much of the material is still unavailable for recovery, and the 65% recovery target cannot be met.
Based on technological, carbon footprint, and recovery concerns with pyro processing, many companies have developed solution-based processes to recover 90%+ of the metals as well as the graphite. In the battery recycling industry, companies with sky-high valuations and ground level revenue streams are common. Companies such as Li-Cycle, Primobus, Northvolt, Lithion, and Ascent Elements have developed hydrometallurgical processes to recover battery metals using low temperature operations. In addition, each of these companies claim they will reach commercial scale within a year or two. TES is the only recycling company to claim that they produce commercial scale, battery grade metals; however, their current EOL LIB process rate is 14 tonnes per year in their Singapore facility from LCO cathode type batteries from a single OEM(Steinbrecher, 2021). Specifically, TES is unable process multiple cathode types or diverse types of LCO battery packs.
Last November, Ascend Elements (formerly known as Battery Resourcers) published an article in Joule demonstrating recycled battery materials from NMC11 meet the same or improved electrochemical qualities over primary production battery metals(Ma et al., 2021). Similarly, Northvolt Ett at Northvolt Labs in Västerås demonstrated that the electrochemical performance of battery cells recycled from NMC battery materials are similar as those originating from mined products(Northvolt Produces First Fully Recycled Battery Cell, 2021). There are two observations about these announcements. The Ascent Elements and Northvolt’s recycled material was made into cathodes and testing was conducting using coin cells. There is certainly a relationship between the performance of a battery material in a coin cell, pouch cell, and cylindrical cell. In addition, there is relationship between the performance of a cylindrical cell and an array of cells in a battery pack—that could be evaluated in an electric vehicle. However, this recycled material was not tested in a cylindrical cell, EV battery pack, or actual electric vehicle—much less tested in real world conditions.
There are many challenges to creating an industrial scale metal processing facility from successful laboratory process. Andrew Miller, from Benchmark Mineral Intelligence, in his “Critical Materials” webinar, mentions that it takes a minimum of 5 years to scale a mining project to commercial production as well as a minimum of three years to move the chemical processing of mined battery metals into commercial scale production for battery grade production (Miller, 2021).
Approximate timeline to progress supply step
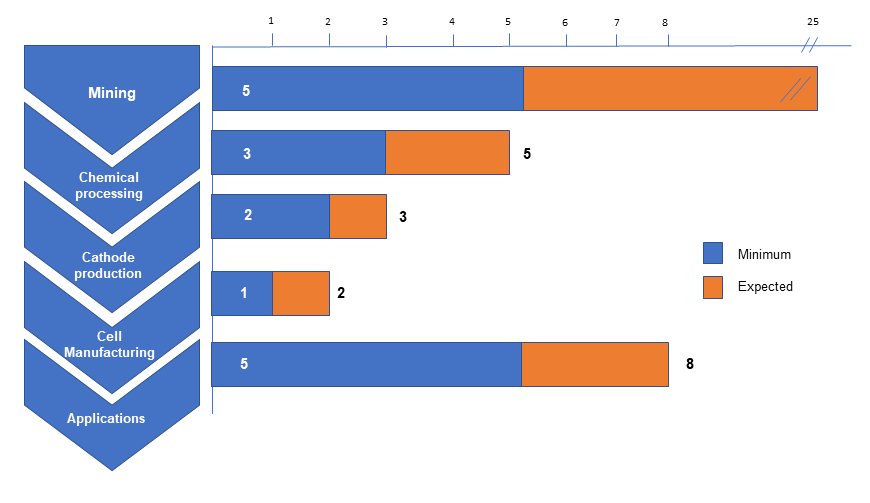
Figure 1: This shows the minimum time required for each stage of the battery value chain (modified from (Miller, 2021)
Qualification into the supply chain will add to these pressures
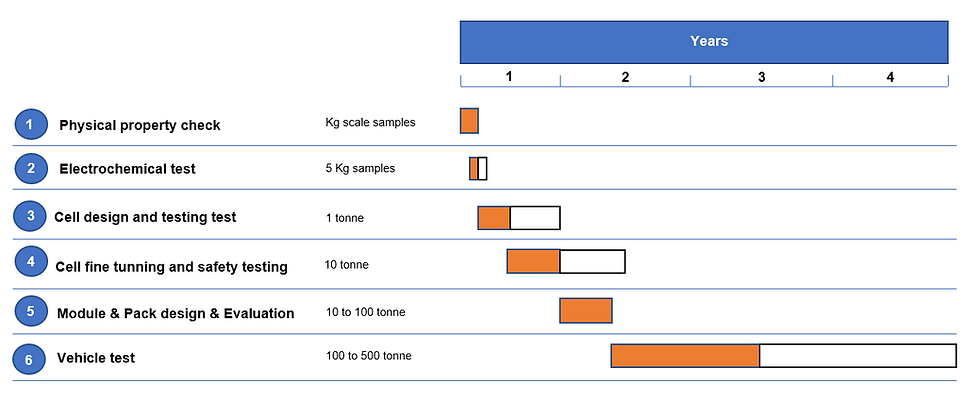
As demonstrated in Figure 2, he states that the qualification process for battery metals is a multi-year process—in addition to the 3-year minimum for scaling the chemical processing.
The “electrochemical test” shown in Figure 2, as Ascent Elements and Northvolt have passed, demonstrates that a battery material is at the early stage of the qualification process. A company passing that test will still have a minimum of several years before that product is accepted by cathode manufacturers.
Similarly, Matthew Fernley of Battery Metals Review voices a similar opinion.
Evolution of Product Testing
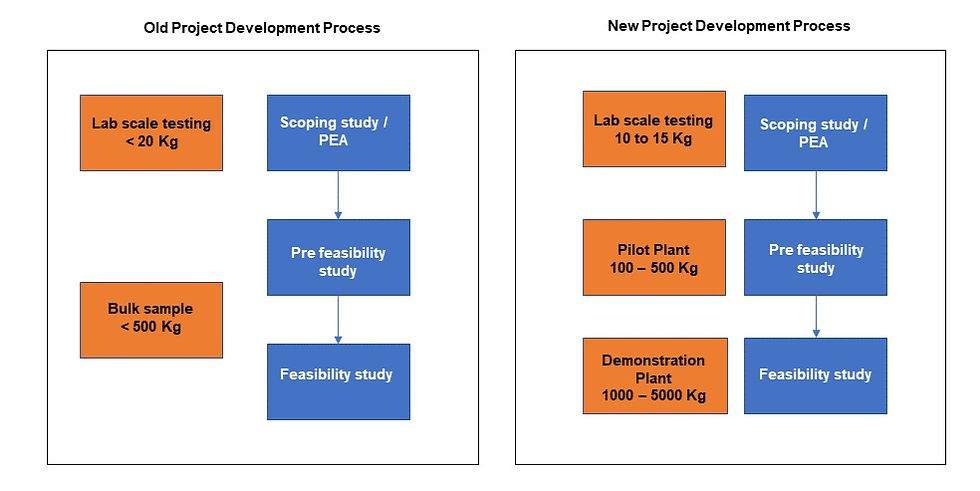
Figure 3: This slide shows the evolution of product testing non-battery metals vs battery grade metals (modified from (Fernley, 2021)
Figure 3: Shows that the stages of product testing for mined products (“Old Project Development Process”) and associated economic studies are different for battery grade mined materials (“New Project Development process”) and require larger product quantities for testing (Fernley, 2021). In addition, the required product standards are more challenging to meet. Figure 4 shows the required elemental product standards for qualified lithium hydroxide and high-purity electrolytic manganese metal. Since all battery metal requirements for OEMs are different, if a project repeatedly fails one aspect of the qualification process (elemental, particle size, electrochemical, etc.) for the material, there may not be another vendor immediately available to accept the current or even an upgraded quality of that material. This adds another layer of risk for a mining or recycling project.
Sample set of battery metal qualification standards
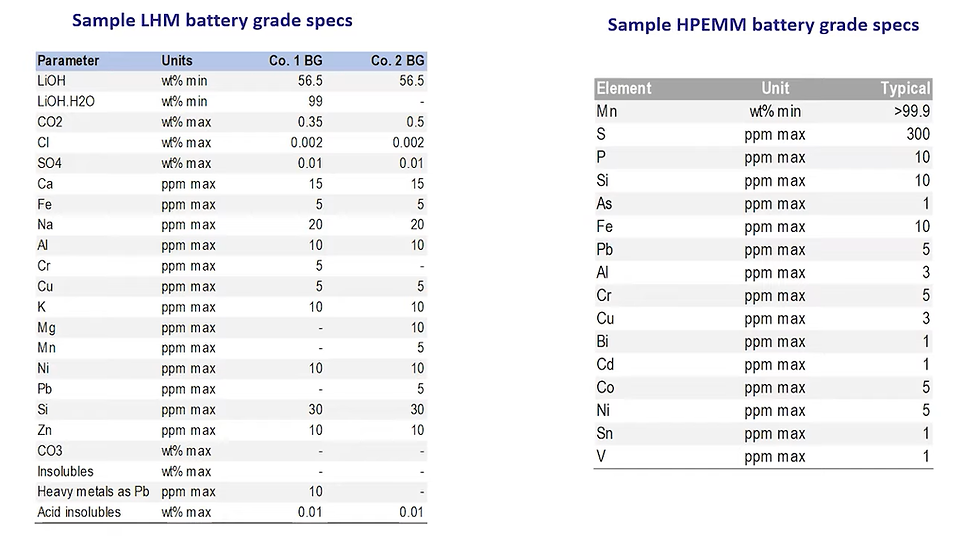
Figure 4: This is a sample set of battery metal qualification standards for an OEM (modified from Fernley, 2021)
Within a mine, there is natural variability in the composition ore of that mine(Goodall, 2021). The basis of geostatistics is that there is certain similarity of ore within a certain distance. The ore block model extended to a 3-dimensional model form the basis for estimates. This association forms the basis of reserve and resource estimating, and the corresponding legal requirements for the economic evaluation of a mining project. Even with high purity and high consistency lithium mines available in Latin America about 20-30% of mines cannot meet battery grade standards for lithium hydroxide and must be converted by a 3rd party to battery grade lithium hydroxide(Fernley, 2021). There is not a similar mechanism to evaluate the economics of a recycling company as there is for a mining company base a recycling company does not own a deposit. Perhaps, a recycling company may a processing technology, but processing technologies are constantly subject to changes in inputs and government regulations.
Nearly all of the upcoming LIB recycling companies claim to be able to recycle all cathode types, cell types, and battery pack types except those, such as Lithion, which cannot recycle LFP batteries.As Figure 5 indicates, the most common installed cathode type is shifting to a LCO rich (cobalt-rich) to more of a NMC811 (nickel-rich) chemistry. There is uncertainty regarding the future composition of installed cathodes both by cathode type and elemental composition.
Cathode shares all Li-ion battery markets based on installed GWh

Figure 5: This shows the installed cathode types in lithium-ion batteries by year (modified from Buechel et al., 2021)
There are techno-economic risks associating with scaling a chemical processing strategy with a discrete cathode chemistry battery to produce battery grade metals from the laboratory to the commercial scale. However, no company can process high volumes of EOL material and battery scrap without accepting a variable cathode chemistry. That chemistry may vary per battery, per shipment, per geography, and per time. This variability does not even include the risk from changing impurity levels from each shipment—which may be the most pertinent risk to producing consistent battery grade metals. Perhaps, the risk could be mitigated by blending shipments from various sources. American Manganese, in market strategy similar to TES, has chosen to partner a tier one manufacturer(s) from feed stock to battery precursor, albeit with more modest ambitions than other companies. However, producing a saleable, consistent quality battery grade output requires consistent input either in a mining or a recycling project.
Variable EOL battery and battery scrap inputs are major risks to commercial battery recycling operations. More importantly, risks to chemical processing scale up and associated required battery metal output are preventing the commercialization of LIB battery recycling. Based on this information, it is important to be critical about the current techno-economic prospects of LIB recycling companies, in the short or medium term, until there is proof of a chemical processing strategy and feed control strategy that produces long-term battery grade materials. Policy makers may have to reconsider their requirements for minimum product rate recycling for LIB and recycling companies should moderate their claims until actual long-term qualification of their battery metals are completed.
Sources
1) Battery Associates. (2021). Roundtable III: Perspectives on the future of batteries.
2) Buechel, C., Bednarski, L., & Wietlisbach, S. (2021). As lithium-ion battery materials evolve, suppliers face new challenges.
3) Cheng, K., Egle, T., & Motter, J. (2021). The Role Of Battery Recycling In The Circular Economy: Part 1 - Key Technologies. Waste 360.
4) EPA. (2021). U.S. National Recycling Goal. https://www.epa.gov/recyclingstrategy/us-national-recycling-goal
5) European Union. (2021). Proposal for a REGULATION OF THE EUROPEAN PARLIAMENT AND OF THE COUNCIL concerning batteries and waste batteries, repealing Directive 2006/66/EC and amending Regulation (EU) No 2019/1020
6) Fernley, M. (2021, October 26). Investing in Junior Battery Metals Companies - Matthew Fernley, Battery Materials Review. 121 Mining Investment TV
Goodall, W. (2021, March 10). Understanding what is feeding your process: How ore variablity costs money. MinAssist.
7) Jacoby, M. (2019). It’s time to get serious about recycling lithium-ion batteries. Chemical & Engineering News, 97(28).
8) Ma, X., Chen, M., Zheng, Z., Bullen, D., Wang, J., Harrison, C., Gratz, E., Lin, Y., Yang, Z., Zhang, Y., Wang, F., Robertson, D., Son, S. B., Bloom, I., Wen, J., Ge, M., Xiao, X., Lee, W. K., Tang, M., … Wang, Y. (2021). Recycled cathode materials enabled superior performance for lithium-ion batteries. Joule, 5(11), 2955–2970.
9) Miller, A. (2021, November). Critical minerals: From discovery to supply chain. Helmholtz Institute Freiberg for Resource Technology.
10) Northvolt produces first fully recycled battery cell. (2021).
11) Rajaeifar, M. A., Raugei, M., Steubing, B., Hartwell, A., Anderson, P. A., & Heidrich, O. (2021). Life cycle assessment of lithium-ion battery recycling using pyrometallurgical technologies. Journal of Industrial Ecology, 25(6), 1560–1571.
12) Sommerfeld, M., Hovestadt, G., & Friedrich, I. H. C. B. (2021). Smelting of Pyrolyzed Lithium-Ion Battery Black Mass using a Calcium-Aluminate Slag System.
13) Steinbrecher, N. (2021, September). 26th International Congress for Battery Recycling (ICBR) 2021.
14) Umicore. (2021). Battery Recycling. https://csm.umicore.com/en/battery-recycling/our-recycling-process/
About the Author
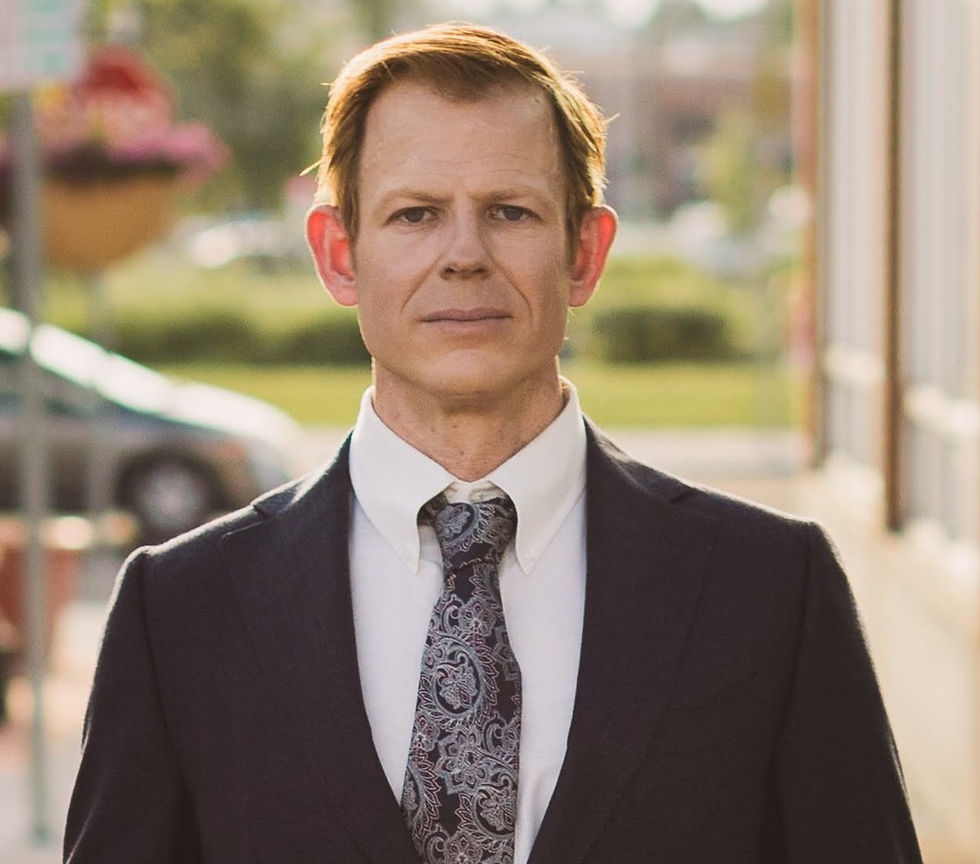
Mark Lawrence Strauss, PhD
Research Engineer, Extractive Metallurgist
Mark Strauss is passionate about creating extractive metallurgical innovations to advance sustainability, clean energy technology, and materials independence. As a post-doctoral fellow at Idaho National Laboratory, he developed innovative recycling strategies to separate and purity lithium batteries into saleable lithium, copper, manganese cobalt and nickel products. His research experience involves the primary and secondary production critical materials using pyro-hydrometallurgical techniques. His undergraduate research involved by-product recovery of rare earths from copper leach solutions at University of Arizona. His masters research in entailed recycling fluorescent lights at Colorado School of Mines. In addition, his PhD graduate research continued to study the separation and purification rare earths from waste fluorescent lights. Strauss graduated in 2018 with a PhD from the Department of Materials Science and Engineering at Worcester Polytechnic Institute.
He is currently developing and scaling electro-hydrometallurgical innovations to improve lithium-ion battery recycling at Aqua Metals Mark traveled last summer throughout Croatia, France, Monaco, Spain, Italy and Germany, by plane, car, bus, bicycle and boat. He is a certified yoga teacher and daily practitioner of mindfulness. On the weekends, he will be bicycling up mountains.
The views expressed in this article are those of the authors alone and not Battery Associates.
Comentarios