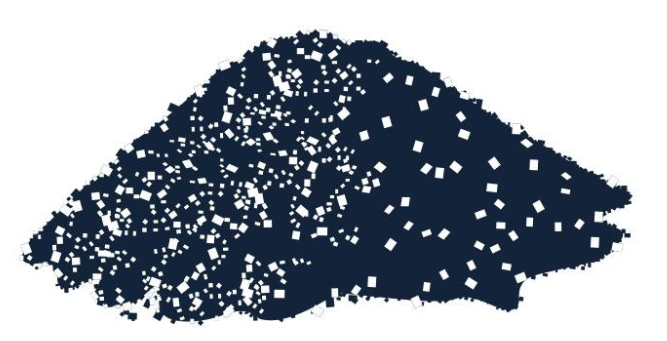
souce: Website
With the population and world economic growth, the global energy demand for power requirements has increased rapidly. Relying on fossil fuels for energy has exacted an enormous toll on humanity and the environment - from air and water pollution to global warming. To address these concerns, sustainable and renewable energy sources, such as solar, wind, and hydro energy have been developed. However, the proliferation of renewable energy is contingent on the development of a sufficient energy storage device in terms of efficiency and cost. The inherently intermittent behavior of renewable energy calls for the development of energy storage devices, which equilibrates the imbalance in supply and demand. Among the various energy storage devices, batteries and supercapacitors potentially unlock and open a new horizon for the energy transition. Although batteries and supercapacitors are both capable of storing energy, the way in which storage occurs is fundamentally different, resulting in several advantages and disadvantages in their performances (Table 1).
Table 1: Performance comparison of supercapacitor and Lithium-ion battery
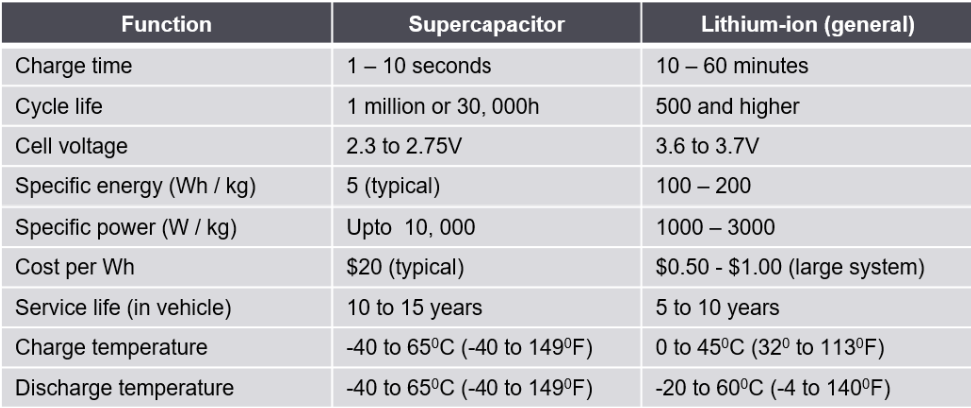
Source: Battery University
Li-ion batteries are more suited for higher energy density applications, meaning it is used for an application where a device needs to meet the long-term energy needs on a single charge. Because of much higher power density than batteries, supercapacitors can provide the quick burst of energy and hence are used for high drain applications making them ideal for powering electric vehicles, stop-start and regenerative braking capabilities. Thus, based on the applications, both supercapacitors and batteries have challenges in terms of energy density, size, lifespan, and capacity. Portable consumer electronics demand Li-ion battery improvement to minimize the size while optimizing performance. The automobile and personal wearable electronics industries are both pushing for the development of batteries and supercapacitors with a longer lifespan and larger capacity as well as lower cost. As a result, extensive research efforts are underway to address these challenges mainly via utilizing more suitable electrodes and electrolytes and introducing different redox-active species. Moreover, several attempts are even made to commercialize these energy storage devices on a wider scale due to the increased focus on safe, efficient, high-performing and cleaner power sources. It has been demonstrated that one way of improving the energy and power properties of batteries and supercapacitors is by increasing the energy storage ability of the electrode materials.
Traditionally, carbon-based electrode materials such as glassy carbon, graphite, and carbon nanotubes are used for energy storage. Electrodes are the conducting materials in a battery or supercapacitor that connect the electrolyte with external wires, to transport current out of the system. The advances in new materials and manufacturing technologies has led to a development of these materials with a wide variety of modifications. Boron doped diamond is one such novel material that has been explored recently as a potential electrode material for batteries and supercapacitors.
Boron-Doped Diamond (BDD)
Diamond is a very intriguing material with extraordinary physicochemical and electronic performance such as hardness, high thermal conductivity, and electrical resistivity, and having a very wide gap semiconductor (5.47 eV at 300 K). Even though diamond has been recognized as a promising material for several applications, its electrically insulating behavior makes it less interesting for electrochemical applications. However, on doping with some definite elements, diamond can behave like conductive materials similar to other large band gap materials, while maintaining their extreme physical properties. Boron or Nitrogen are currently used as dopants for diamond. However, doping using boron is preferred over nitrogen owing to its lower activation energy (0.37 eV for boron and 1.7 eV for nitrogen).
Though BDD obtained by using boron as a dopant has a character similar to that of a regular diamond, it also possesses excellent characteristics of a semiconductor such as low resistance, good thermal conductivity, and higher oxidizing temperature. In addition, they exhibit attractive properties that are useful for many electrochemical processes such as
low background currents,
reduced fouling,
wide electrolytic window (~3 to 3.5 V for aqueous, ~5 to 7.5 V for non-aqueous),
high heat resistance (up to 250℃),
chemical inertness,
high wear and impact resistance and
biocompatibility.
BDD for batteries and supercapacitors
Rechargeable batteries such as lithium-ion (LIB) and supercapacitors are becoming prominent sources for providing portable energy to modern mobile society. The new-generation 3C (computer, communication, and consumer electronics) market demands energy storage devices with lighter weight, higher energy/power density, and longer cycle life. Commercial Li-ion batteries consist of four main parts: electrodes such as anode and cathode, separator, and electrolyte. When Li-ions travel through the electrolyte from one electrode to the other, it facilitates an electric current in the external circuit, powering an electric device. The chemical interaction of these components directly influences the quality and performance of the battery. Although Li anode has a superior theoretical capacity (3.862 mAh g−1) and a high redox potential, it possesses several problems like dendrite and poor cyclability. As a result, large research efforts have been focused on the development of graphitic carbon or lithium metal alloys as an anode. However, all these materials suffer morphological changes during the charge-discharge cycling, which in turn results in very poor cycle life. The use of BDD electrode materials, which have very stable surfaces and excellent electrochemical properties when compared to other carbon forms like glassy carbon and highly oriented pyrolytic graphite, has been proposed as an alternative material for battery applications.
BDDs are commonly fabricated using a chemical vapor deposition (CVD) method. The operating conditions such as temperature, pressure, and deposition time impact the characteristics of the final product. Besides CVD conditions, the sp3/sp2 ratio and the surface termination had a huge impact on the final properties of BDD. Several attempts were reported on the use of boron-doped diamonds in batteries. They mainly include:
Incorporation of Li in the diamond during CVD growth
Lithium-ion implantation into BDD and using it as an anode
Lithium-ion diffusion with and without an electrical field
BDD used as cathode where Li foil is anode
Changing doping levels for lithium intercalation in BDD
Depending on the manufacturing and operating conditions, these studies had varying results in terms of the diffusion rate of Li ions, reversible capacity, and charge-discharge efficiency. It was usually observed that the presence of boron is useful to stabilize Li-ions since the Li 2s electrons can be easily transferred to the empty states associated with the dopant. The binding energy of Li atoms is highly increased, preventing, therefore, clustering of Li species, which is also an important requirement for practical applications. Overall, the use of boron-doped diamonds in batteries resulted in improved electrochemical performance and increased efficiency and longevity compared to traditional batteries when the integration of lithium was successful.
Similar to batteries, scientists also explored the idea of using boron-doped nanodiamonds as electrodes in supercapacitors. The wide potential window of boron-doped diamonds enabled the development of a high-energy storage device with good stability. Even the basic supercapacitor with BDD electrodes produced much higher voltage than conventional cells, resulting in much higher energy and power densities for the supercapacitor and high stability even after 10,000 cycles of charging and discharging.
Though utilization of BDD for batteries and supercapacitors is promising, considering the number of reports published so far, further research and development should be carried out to ensure effective application and exploitation of BDDs as electrode materials for energy storage.
Challenges of BDD for Energy Storage
Supply Chain and Manufacturing Process: Main challenges with BDD batteries arise during the manufacturing process. Chemical vapor deposition (CVD) and sub-CVD are processes in which gasses at extremely high temperatures force carbon to crystallize on a substrate material. They are not only very expensive but also energy-intensive, which remains a bottleneck in diamond production. Furthermore, incomplete cleaning of the diamond surface, choice of material to grow boron-doped diamonds, and unsuitable fabrication conditions are some of the factors that make the use of BDD challenging in energy storage devices, especially batteries. The supply chain shortages for substrates and lithium, a limited number of production methods, and complex manufacturing equipment also do not help the emergence of BDD-based batteries.
Cost: Global BDD electrode market is anticipated to rise at a considerable rate between 2022 and 2028. However, the cost of CVD processes and raw materials is also on the rise. Even though the utilization of BDD in water treatment and other electroanalytical applications may not be impacted, given the limited number of BDD manufacturers and the rise in lithium and substrate material prices, it would be safe to say that the use of boron-doped diamond in batteries does not seem feasible in the near future compared to their advantages. On the other hand, for supercapacitors, it is possible that diamonds can drive our electronic and physical lives in the near future.
Battery Analytics: Although the number of studies focusing on the BDD for batteries and supercapacitors has been increasing since the beginning of the 2000s, most studies stay within the lab scale. Further research and developments should be focused on understanding and quantifying the relationship of the properties of the BDD materials, such as sp2-type carbon content, grain boundary size (micro, and nanodiamond), level of doping, diamond conductivity, surface termination, etc, on the lithium intercalation behavior are still required for further practical development.
Regulations and Policies: As BDD is not prevalent enough for commercial use of energy storage, there are not any known regulations regarding them in the battery industry. However, with the need for urgent and more intensive actions against climate change, the profound ambition of most countries is to build a clean-energy economy with a circular, responsible and just battery value chain. By 2030, countries like Europe, the United States and their partners are planning to establish a secure battery materials and technology supply chain which can support long-term economic competitiveness, create equitable clean-energy manufacturing jobs, and enable decarbonization. Some of the goals for achieving these visions include (1) securing access to raw and refined materials and discovering alternatives for critical minerals for commercial and defensive application, (2) stimulating the US and Europe electrode, cell and pack manufacturing sectors through in-house processing and production, (3) enabling a secure end-of-life reuse and critical materials recycling ecosystem and (4) supporting scientific R&D to advance battery technology. The overall goal is to create a competitive and sustainable battery manufacturing industry and reduce the battery manufacturing dependence on scarce materials. Boron doped diamonds can provide benefits for a robust, secure, and domestic industrial base for batteries. Due to their extraordinary physicochemical and electronic performances, the BDD can be substitutes that are sustainable with increased energy density and may reduce recycling needs. Though the markets are promising in terms of their end-use applications and requirements, the use of BDD in batteries still needs a lot of innovation and research and development (R&D) to meet the needs of the growing market. On the way forward, with the introduction of clear policies, especially that support further R&D can play a critical role in achieving diamond enabled energy storage with reduced cost and improved performance.
End of Life: Even rechargeable batteries degrade over time and ultimately cease to work. The end of life of an energy storage device is when they have reached the end of their usefulness and/or lifespan and no longer operate at sufficient capacity. It is usually determined in terms of cycle life and total energy throughput. The cycle life is the total number of times that a battery can be charged and discharged, whereas total energy throughput is the total amount of energy that will pass through the battery and supercapacitor over the course of their lifespan. Integration of BDD in batteries and supercapacitors is anticipated to increase both the cycle life and the total energy throughput. However, commercial-scale studies are needed to have a more realistic understanding of total energy throughput. Despite no longer operating at peak performance, end-of-life energy storage devices, for example batteries, can still hold 70-80% of their initial capacity. These batteries have the immense potential to be repurposed into second-life batteries for use in less demanding applications (i.e. stationary energy storage). When a battery cannot be reused in secondary application, they need to be recycled, which allows the recovery of as many critical elements as possible. However, more R&D needs to be performed to carefully understand the end-of-use and recycling of BDD based batteries, which is crucial for a stable supply chain.
Sources
BU-209: How does a supercapacitor work? (2021). Battery University. Retrieved from https://batteryuniversity.com/article/bu-209-how-does-a-supercapacitor-work on April 2022.
Xu, J., Yang, N., Heuser, S., Yu, S., Schulte, A., Schönherr, H., & Jiang, X. (2019). Achieving ultrahigh energy densities of supercapacitors with porous titanium carbide/boron‐doped diamond composite electrodes. Advanced Energy Materials, 9(17), 1803623.
Supercapacitors vs. Batteries. (2019). Kemet Engineering Center. Retrieved from https://ec.kemet.com/blog/supercapacitors-vs-batteries/ on January 10, 2022.
Gao, F., & Nebel, C. E. (2016). Diamond-based supercapacitors: realization and properties. ACS Applied Materials & Interfaces, 8(42), 28244-28254.
McCreery, R. L. (2008). Advanced carbon electrode materials for molecular electrochemistry. Chemical reviews, 108(7), 2646-2687.
Muzyka, K., Sun, J., Fereja, T. H., Lan, Y., Zhang, W., & Xu, G. (2019). Boron-doped diamond: Current progress and challenges in view of electroanalytical applications. Analytical methods, 11(4), 397-414.
Balmer, R. S., Brandon, J. R., Clewes, S. L., Dhillon, H. K., Dodson, J. M., Friel, I., ... & Woollard, S. M. (2009). Chemical vapour deposition synthetic diamond: materials, technology and applications. Journal of Physics: Condensed Matter, 21(36), 364221.
Einaga, Y., Foord, J. S., & Swain, G. M. (2014). Diamond electrodes: diversity and maturity. Mrs Bulletin, 39(6), 525-532.
Einaga, Y. (2010). Diamond electrodes for electrochemical analysis. Journal of applied electrochemistry, 40(10), 1807-1816.
Brillas, E., & Mart, C. A. (Eds.). (2011). Synthetic diamond films: preparation, electrochemistry, characterization, and applications (Vol. 8). John Wiley & Sons.
Fujishima, A., Einaga, Y., Rao, T. N., & Tryk, D. A. (Eds.). (2005). Diamond electrochemistry. Elsevier.
Xu, W., Wang, J., Ding, F., Chen, X., Nasybulin, E., Zhang, Y., & Zhang, J. G. (2014). Lithium metal anodes for rechargeable batteries. Energy & Environmental Science, 7(2), 513-537.
Kong, L., & Pecht, M. (2020). A look inside your battery: Watching the dendrites grow. Battery Power. Retrieved from https://www.batterypoweronline.com/news/a-look-inside-your-battery-watching-the-dendrites-grow/ on April 29, 2022.
Bates, A. M., Paxton, W. F., Spurgeon, J. M., Park, S. D., & Sunkara, M. K. (2021). Earth-abundant redox couples using durable boron doped diamond electrodes: Beyond vanadium redox couples. Applied Energy, 282, 116252.
Almeida, E. C., Trava-Airoldi, V. J., Ferreira, N. G., & Rosolen, J. M. (2005). Electrochemical insertion of lithium into a doped diamond film grown on carbon felt substrates. Diamond and related materials, 14(10), 1673-1677.
Ferreira, N. G., Mendonça, L. L., Airoldi, V. J. T., & Rosolen, J. M. (2003). Electrochemical intercalation of lithium into boron-doped CVD diamond electrodes grown on carbon fiber cloths. Diamond and related materials, 12(3-7), 596-600.
Rehnlund, D., Lindgren, F., Böhme, S., Nordh, T., Zou, Y., Pettersson, J., ... & Nyholm, L. (2017). Lithium trapping in alloy forming electrodes and current collectors for lithium based batteries. Energy & Environmental Science, 10(6), 1350-1357.
Christy, A. Y. M. T., Nahm, K. S., Hwang, Y. J., Suh, E. K., Kulandainathan, M. A., Premkumar, T., & Stephan, A. M. (2008). Lithium insertion studies on boron-doped diamond as a possible anode material for lithium batteries. Ionics, 14(2), 157-161.
Wang, J., He, Z., Tan, X., Wang, T., He, X., Zhang, L., ... & Du, K. (2020). Hybrid supercapacitors from porous boron-doped diamond with water-soluble redox electrolyte. Surface and Coatings Technology, 398, 126103.
Boron Doped Diamond Electrode Market 2022. (2022). SNN News. Retrieved from https://www.snntv.com/story/46076018/Boron-Doped-Diamond-Electrode-(BDD)-Market on April 2022.
National blueprint for lithium batteries - executive summary 2021-2030. (2021). Federal Consortium for Advanced Batteries.
About the author
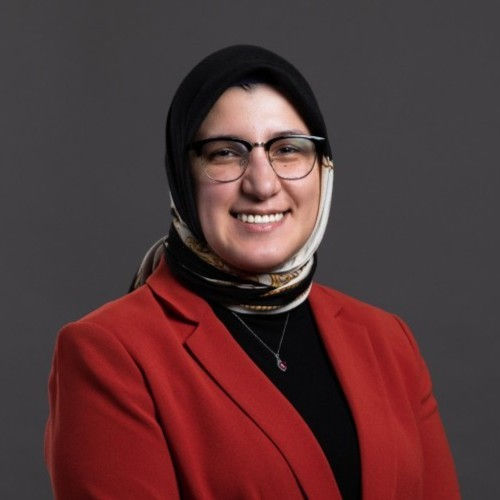
Humeyra B. Ulusoy Erol, Ph.D
Senior research engineer at CatalyzeH2O
Dr. Humeyra B. Ulusoy Erol is currently a senior research engineer at CatalyzeH2O located in Fayetteville, Arkansas. She is responsible for product design, research, and development of electrochemical techniques and solving technical challenges for the removal of explosives and other contaminants from wastewater. She was previously an electrochemistry engineering manager at Weo in Miami, Florida, where she worked closely with executive, engineering, marketing, product, and sales teams. Humeyra received her Ph.D. from the University of Arkansas where she collaborated with industry partners/sponsors to solve industry-related water treatment problems as part of the MAST center. She is based in Fayetteville, Arkansas, USA. Humeyra chose to join the BatteryMBA programme to stay up-to-date and develop her skillset in energy and battery industries, grow her network of battery leaders as well to learn from and eventually be part of battery industry leaders.
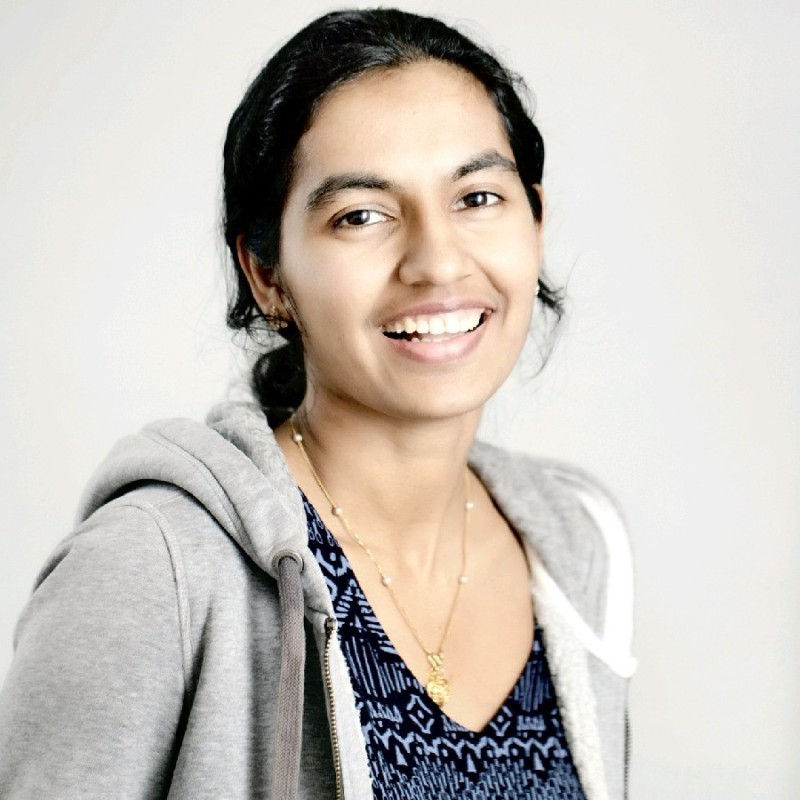
Anjali A. Edathil
PhD student - Technical University Denmark
Anjali A Edathil, is a final year PhD student at the Technical University Denmark researching on freestanding 3D sustainability energy materials as part of the PHEONEEX project funded by the European Research Council. Before her PhD she completed a Master of Science in ChemicalEngineering from Khalifa University UAE and Bachelor's degree in Polymer Engineering from Amrita University, India. As a true material science enthusiast her interest lie in utilizing sustainable biopolymers to solve energy and environmental challenges and thus accelerate sustainable energy revolution.
The views expressed in this article are those of the authors alone and not Battery Associates.
Comments